Soil Ecologist, Ph.D.
We often don't think much about the ground that we walk on, but soils contain the highest biodiversity on Earth!
Just half a spoonful of soil can contain
1 million species of bacteria and
200 meters of fungal hyphae!
Soil is a difficult habitat to explore because much of the soil biome is invisible to the naked eye. Fortunately, advances in genomic sequencing have made it possible to uncover the immense diversity of soil microbial communities. But harnessing this enormous amount of information in a way that is meaningful is challenging.
In my work, I use a combination of tools including field soil collection, glasshouse and laboratory experiments, genomics, and bioinformatics to identify the relevance of soil microbiome complexity for ecosystem and plant function.
Ultimately, my goal is to conduct rigorous and informative science for real-world environmental challenges.
CURRENT WORK
Soil microbial carbon cycling dynamics of grasslands in response to
land management and restoration
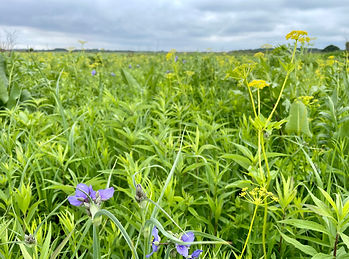


Image by Cooper Rosin
Over 90% of North America's tallgrass prairie was lost due to widespread conversion to row-crop agriculture. It is estimated that grasslands cover nearly 40% of the Earth's land surface and store over one-third of the world's terrestrial carbon stock, so restoring grassland ecosystems like tallgrass prairie is integral to mitigating atmospheric carbon emissions.
Though microscopic and often invisible, microbial communities in soil are one of the key determinants of terrestrial carbon cycling. Microbes release carbon as carbon dioxide and methane by consuming and subsequently decomposing organic carbon inputs from plants. Conversely, microbes stabilize carbon when some of the inputs are converted into microbial residues, including metabolites and dead microbial biomass which adhere to mineral soil surfaces and become resistant to decomposition. This ratio of carbon stored in microbial biomass relative to carbon respired is known as carbon use efficiency and is an important indicator of soil organic carbon content. Accumulating evidence suggests that microbial functional groups and taxa vary in carbon use efficiency, so environmental factors that shape soil microbial communities may have consequences for soil carbon stocks.
My research with the Barber lab at San Diego State University examines potential carbon use efficiency of bacterial and fungal soil communities and degradation rates of several forms of organic carbon among never-cultivated remnant tallgrass prairie and previously cultivated, restorted tallgrass prairie to assess how grassland land management influences microbial carbon cycling dynamics.
Read more about this work here.

Fire-induced changes to soil microbial communities and the consequences for carbon cycling
Across the globe, fire events are becoming more frequent with climate change, which can have important consequences for soil communities and carbon cycling. My colleagues and I are seeking to identify how fire-induced changes to bacterial and fungal community composition may influence carbon cycling.
Using soils collected from a 2016 wildfire in Great Smoky Mountains National Park, Tennessee, we conducted amplicon sequencing and measured soil abiotic characteristics to assess the composition of soil microbial communities and ecosystem functions associated with carbon cycling. We are seeking to identify: 1) if fire shifts microbial community composition to a community comprised of bacterial and fungal taxa that have relatively slow metabolic life histories (slow-growing, thrive under low resource conditions) and therefore high carbon use efficiencies (CUE), and 2) if post-fire shifts in microbial CUE membership are associated with differences in microbial biomass carbon and the activity rates of multiple carbon-degrading extracellular enzymes.

PREVIOUS WORK

Fire-induced changes to soil microbial communities and the consequences for restoration of a keystone tree species
Oaks are a keystone tree species of North American hardwood forests, but oak-dominated forests are in decline due in large part to exclusion of frequent, low-severity fire, a necessary disturbance for fire-dependent tree species like oaks. Oak regeneration benefits from prescribed burning and reduced competition with fire-intolerant tree species. Despite recommendations for research on the role of the microsite environment for oak regeneration, very little is known about the interacting effects of fire, soil, and the surrounding plant community on oak establishment.
Using a wildfire system in Great Smoky Mountains National Park in Tennessee, my colleagues and I examined the bacterial and fungal community composition of burned and unburned soils post-fire. We conducted a glasshouse experiment and grew black oak (Quercus velutina) seedlings in treatments of plant neighbor (oak vs. pine seedling) and soil burn status (unburned vs. burned soil) to assess the effects of plant community, fire-induced shifts in soil microbial communities, and their interaction on oak seedling growth.

We found that fire reduced the diversity and relative abundance of plant pathogenic fungi, but did not affect soil bacterial communities. Shifts in soil fungal community composition enhanced oak seedling root growth, but the effect of the soil microbiome was mediated by plant neighbor interactions. Oak seedling root growth was negatively correlated with diversity of plant pathogenic fungi. Root growth was enhanced in burned soil relative to unburned soil, but only when growing with a pine seedling neighbor as opposed to an oak seedling neighbor. Results from this study show that interactions between soil microbes and nearby plants can in part mediate oak seedling growth. As such, nuanced decisions that consider the ecological interactions of the microsite environment are needed to achieve desired outcomes for oak regeneration.
Read more about this work here.

Soil microbial mediation across the plant phenotype spectrum
While distinct soil microbiomes and individual soil microbial taxa can alter particular plant traits under highly controlled conditions, little is known about the role of particular microbial taxa and microbial functions within complex soil microbial communities for mediating plant phenotypes or if the strength of microbial mediation of plant phenotype varies among plant species or plant phenotypic traits. Examining how the plant phenotype spectrum is influenced by the taxonomic and functional composition of complex soil microbial communities allows for a more accurate understanding of the biotic environmental drivers of plant phenotype.
Using rhizosphere soil collected from natural field sites in eastern Tennessee, I conducted a microbiome transfer glasshouse experiment to assess if the taxonomic and functional composition of different soil microbiomes would differentially shift growth, physiological or reproductive traits of three Solidago species, a common herbaceous plant genus native to North America.
We found that soil microbiome inoculations influenced Solidago growth traits more than physiological and reproductive traits. Root growth of one of the Solidago species was negatively correlated with 77% of the indicator bacterial and fungal taxa from one of the soil microbiome treatments. This study shows that soil microbial mediation of plant phenotype varies by plant traits, is not universal across plant species, and can be associated with a small number of microbial taxa.
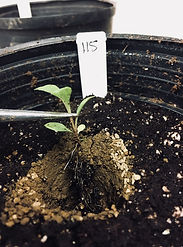
This research illustrates that specific microbial taxa within a soil microbiome are associated with shifts in plant phenotype by pinpointing important individual microbial taxa from complex field soil microbial communities.

Interactions of soil microbiomes and plants vary in response to environmental gradients
Research on plant-soil feedbacks (PSF) has largely been undertaken in highly controlled greenhouse conditions and has established that plant species differentially alter abiotic and biotic soil conditions that in turn affect growth of other conspecific and heterospecific plants in that soil. Yet, whether PSFs under controlled greenhouse conditions reflect PSFs in natural environments where plants are exposed to a range of abiotic and biotic pressures is still unresolved.
To address how environmental context affects PSF, my colleagues and I conducted a meta-analysis of previously published studies that examined plant growth responses to multiple forms of competition, stress, and disturbance across various PSF designs. We sought to determine the following: 1) can competition, stress, and disturbance alter the direction and/or strength of PSFs? and 2) do particular types of competition, stress, or disturbance affect the direction and/or strength of PSFs more than others?
We discovered that relatively little is known about how PSFs respond to environmental stress and disturbance compared to plant-plant competition. Secondly, specific environmental stressors enhanced positive effects of soil microbes on plant growth, and this was driven by drought stress. Thirdly, PSFs varied widely within environmental disturbance treatments, likely because very few PSF studies have been conducted in the context of environmental disturbance. This work highlights that there is a significant gap in our scientific understanding of how soil microbial communities and plants interact under environmental disturbance. As climate change increases the frequency and severity of environmental disturbances worldwide, much of our research moving forward should be aimed at examining how these important soil microbial-plant interactions function under climate change scenarios.
Read more about this work here.
Art by Brooke Weiland Studios
Photography by Kendall Beals